Patterns of Failure in Deep Venous Arterialization and Implications for Management
Naveed S. Zaman, BS1; Christopher Shackles, DO, MPH2; Kevin T. Moriarty, BA, BSN, RN3; Kevin Herman, MD2; John H. Rundback, MD2
ISSN: 2694-3026
J CRIT LIMB ISCHEM 2022;2(3):E58-E63. Epub 2022 July 27. doi: 10.25270/jcli/CLIG22-00008
Abstract
Objective. The primary objective of this study was to describe a novel classification system to characterize patterns of failure in deep venous arterialization (DVA) and suggest intervention strategies that correlate with these patterns. Methods. This was an observational case series with retrospective chart and radiology image review. Data including time between initial DVA and reintervention, location of failure relative to DVA site, and corresponding reintervention strategies were recorded. The primary endpoints measured were the frequency of failure patterns and reintervention strategies employed. Results. Sixty-seven DVAs were performed in this cohort using commercially available devices. Twenty-five cases (37.9%) required reintervention. From the reintervention cases, two-thirds presented with multiple failure patterns simultaneously; 68.0% of repeat cases involved venous outflow failure while 56.0% of cases involved arterial inflow lesions. Strategies used in our cohort of 23 reintervention cases included drug-coated balloon or percutaneous transluminal angioplasty (72.0%), additional stent deployment (60.0%), or additional graft placement (8.0%). Discussion. Based on the results, we propose a classification of “failure type” patterns as one or a combination of the following based on the occlusion site found during reintervention, including arterial inflow, intragraft, transitional vein (proximate to distal edge of stent graft), venous arch, and/or undefined. In these cases, angioplasty, additional stent deployment, and/or additional graft placement can be used in reintervention according to our model. Conclusion. DVA failures and reinterventions are common. It is possible to identify patterns and related treatments using our classification system. Further studies are needed to validate our model.
J CRIT LIMB ISCHEM 2022;2(3):E58-E63. Epub 2022 July 27. doi: 10.25270/jcli/CLIG22-00008
Key words: deep venous arterialization, reintervention strategy
Introduction
Deep venous arterialization (DVA) is a procedure used to treat critical limb ischemia (CLI) patients who have had little to no success with prior interventions and lack further endovascular treatment options (“no-option” or “nonreconstructible” disease patterns). CLI usually presents as stage 4-6 on the Rutherford classification of peripheral arterial disease (PAD), with severe occlusion of leg/foot arteries, claudication, and gangrene or necrosis.1,2 In these patients, if there is no endovascular option or surgical target or conduit for bypass, major above-ankle amputation has historically been required, which can present with further complications and is associated with earlier mortality.3 DVA provides a revascularization alternative in “no option” patients who are otherwise facing amputation. The procedure involves creating a connection between a site of arterial inflow and a deep venous outflow target with the intent of establishing retrograde venous flow to bypass arterial occlusion and provide pedal perfusion.4
A 2018 meta-analysis of 15 articles concerning 768 patients who underwent venous arterialization found a 75% 1-year limb salvage rate.5 The same analysis reported that 6 studies found a range of 59%-71% 1-year DVA patency. The ALPS multicenter study, which looked at 32 DVA patients using a proprietary catheter DVA system (LimFlow SA) across 4 centers found that 71% of patients had 1-year amputation-free survival (AFS), with 68.2% 1-year wound healing.6 The PROMISE I trial using the LimFlow system found that, for a cohort of 32 patients, 70% and 75% of patients experienced 1-year AFS and wound-healing, respectively.7 However, reintervention was also common, with 52% of patients in the PROMISE I trial requiring reintervention.7 In the ALPS study, 54.8% of patients underwent repeat procedures,6 with 67% of all patients in the study suffering an occlusion following DVA. According to a separate study by Huizing et al, 74% of DVA patients from the Northwest Clinics (the Netherlands) and Changi General Hospital (Singapore) developed restenosis.8
Although there are proposed models to estimate or predict the timing and probability of restenosis or occlusion in DVA patients,8 patterns of stenosis localization resulting in DVA conduit failure have not been described. These patterns may be relevant to management strategies at the time of clinically driven reintervention. A pattern-based model can thus improve the success of reinterventions by providing a framework for management strategies in various reintervention scenarios. We propose a five-tier classification system for patterns of DVA failure that relates restenosis to anatomical location and/or positional relation to implanted devices. Furthermore, we describe possible management strategies for reintervention that correlate with these patterns of occlusion.
Methods
Indications for initial DVA and reintervention. All patients had “no-option” disease characterized by chronic total occlusion, prior failed endovascular and/or open surgical procedures, and an angiographic absence of reconstituted arteries in the lower leg or foot suitable for surgical bypass. Optimized medical therapy with moderate-to-high dose statins, antiplatelets with or without Factor Xa inhibitors, and cilostazol was utilized, if possible, in all cases prior to and following DVA. Patients qualified as reintervention cases if restenosis or occlusion of the DVA was discovered on clinical or duplex surveillance and DVA reintervention deemed clinically necessary by the attending physician.
The initial DVA was performed using “off-the-shelf” devices. An arteriovenous conduit was established by using an Outback system (Cordis) to allow puncture from the donor tibial artery into an inflated balloon positioned in the adjacent vein via pedal or lower tibial venous access. Alternatively, a balloon was inflated in the donor tibial artery as a target for puncture via an Outback inserted retrogradely via the pedal venous access. DVA was completed with angioplasty, balloon valvulotomy, and placement of a 5-mm-diameter Viabahn stent-graft (Gore) of a length sufficient to cross the initial arteriovenous fistula and to extend below the lowest visualized tibial venous valve if possible. Duplex ultrasound surveillance was performed within 2 weeks and then monthly thereafter, with shorter interval evaluation if the patient presented with ongoing or worsening ischemia, wounds, or gangrene and clinical hand-held doppler examination suggested DVA dysfunction. Reintervention was performed for applicable cases involving restenosis or occlusion, and utilized percutaneous transluminal angioplasty (PTA)/drug-coated balloon angioplasty (DCB), additional stent-graft placement or venous Supera woven nitinol stents (Abbott Vascular), and/or retrograde venous access as necessary to allow successful recanalization of the DVA.
Data collection. A retrospective patient chart and angiographic image review was performed for all DVA procedures performed by 2 operators in both a single community hospital and an office-based laboratory setting between January 2018 and July 2022. Patient demographics including initial DVA date, anatomical location of DVA, date of reintervention, time between initial and reintervention procedures, location of failure relative to DVA site, and corresponding reintervention strategies were recorded. Patients that underwent below-knee or above-knee amputation at any point during the study were considered clinical failures and excluded from the cohort. The primary outcome measured was the frequency of each pattern of DVA failure as identified at the time of reintervention, as well as the specific reintervention strategies employed.
Classification of DVA failure types. Image review of reintervention procedures allowed for identification of sites of stenosis or occlusion contributing to DVA failure. Based upon these findings, we propose a classification of disease patterns based upon sites of occlusion or stenosis contributing to DVA failures (Figure 1). In this classification system, a pattern is characterized as a “failure type.” Type 1 involves occlusions that affect arterial inflow, including lesions proximal to the DVA site (1a) or at the proximal stent edge of the DVA (1b). Type 2 involves occlusions within the graft. Type 3 characterizes occlusions that affect venous outflow, including lesions at the DVA distal stent edge (3a), within transitional veins (medial/lateral plantar veins) (3b), or within previously placed stents in the transitional veins (3s). Type 4 involves lesions of the venous arch of the foot, specifically a recurrent absence of filling of the entire pedal arch that was patent at the time of the initial DVA completion. Type 5 cases are “undefined” because the exact location of the culprit lesion(s) causing DVA failure could not be clearly identified. Although distinct type and subtype patterns have been delineated, it is possible for DVA failures to concurrently exhibit multiple failure types.
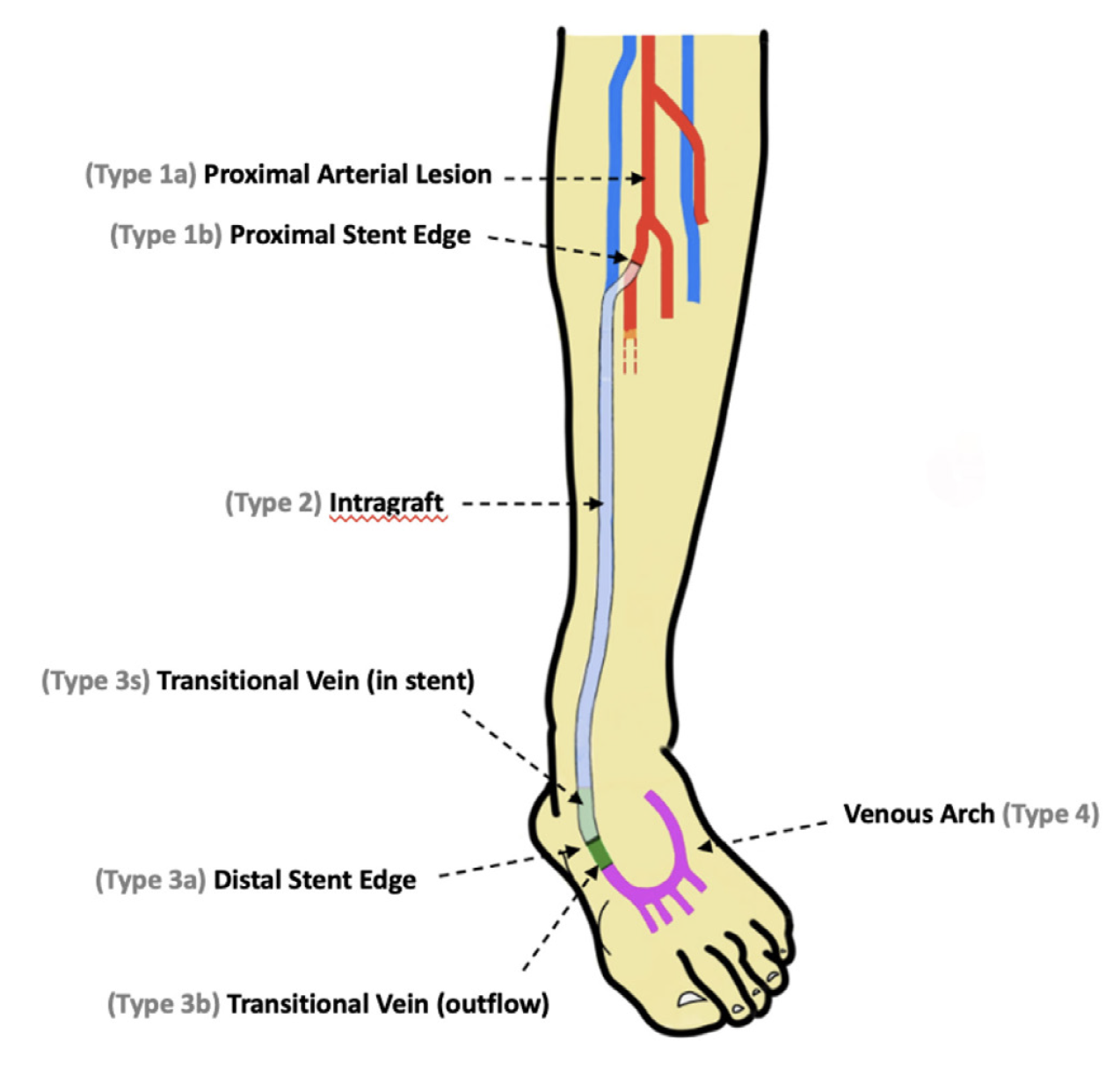
Results
Sixty patients were identified in this study with a total of 67 successful DVAs performed in the cohort (Table 1). Twenty-five DVAs underwent clinically driven reintervention. Four of these patients had 2 separate reinterventions of the same DVA and thus were counted as 8 separate reintervention cases. In total, 25 cases (37.3%) of our original 67 DVAs qualified as reinterventions. Of these cases, 80.0% of reinterventions occurred in posterior tibial DVAs while 20.0% occurred in anterior tibial DVAs. The average time between initial DVA and subsequent reintervention was 111 days. There was high variability in time between the initial DVA and subsequent reintervention, ranging from 2 to 329 days.
The frequency of DVA failure types in our cohort is presented in Table 2. Examples of angiography imaging for each failure type are presented in Figures 2-6. The frequencies of Type 1a and Type 1b failures (Figure 2) were 44.0% and 16.0%, respectively. A Type 2 intragraft failure was demonstrated in 32.0% of the cohort (Figure 3). Among the venous outflow lesions, 24.0% were Type 3a, 24.0% were Type 3b, and 8.0% were Type 3s (Figure 4). Fifty-two percent of cases demonstrated a Type 4 failure of the venous arch (Figure 5). Four percent of reinterventions were Type 5 undefined. Two-thirds of the reintervention group presented with multiple failure types concurrently (Figure 6). Sixty-eight percent of repeat cases were due to lesions in the venous outflow (Types 3 and 4), and 56.0% of cases demonstrated arterial inflow lesions (Type 1).
Strategies used in reintervention cases were determined by the operating physician at the time of reintervention and are summarized in Table 3. Across the reintervention group, strategies and their respective frequency of use primarily included PTA/DCB (72.0%), additional stent deployment (60.0%), or additional graft placement (8.0%). Overnight catheter-directed thrombolysis was utilized in 2 patients. Multiple strategies were used in a patient as necessary to treat all identified patterns of failure.
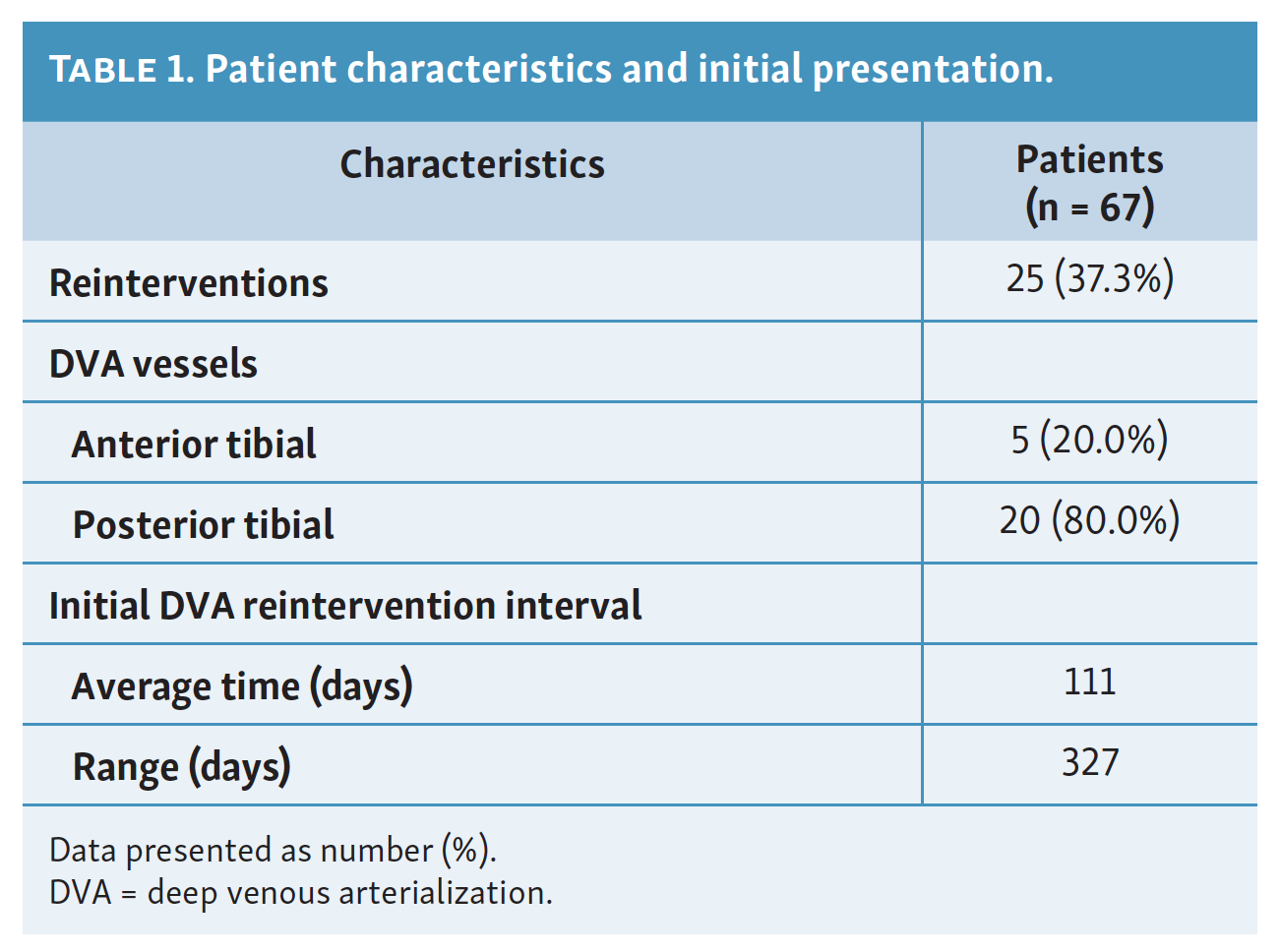
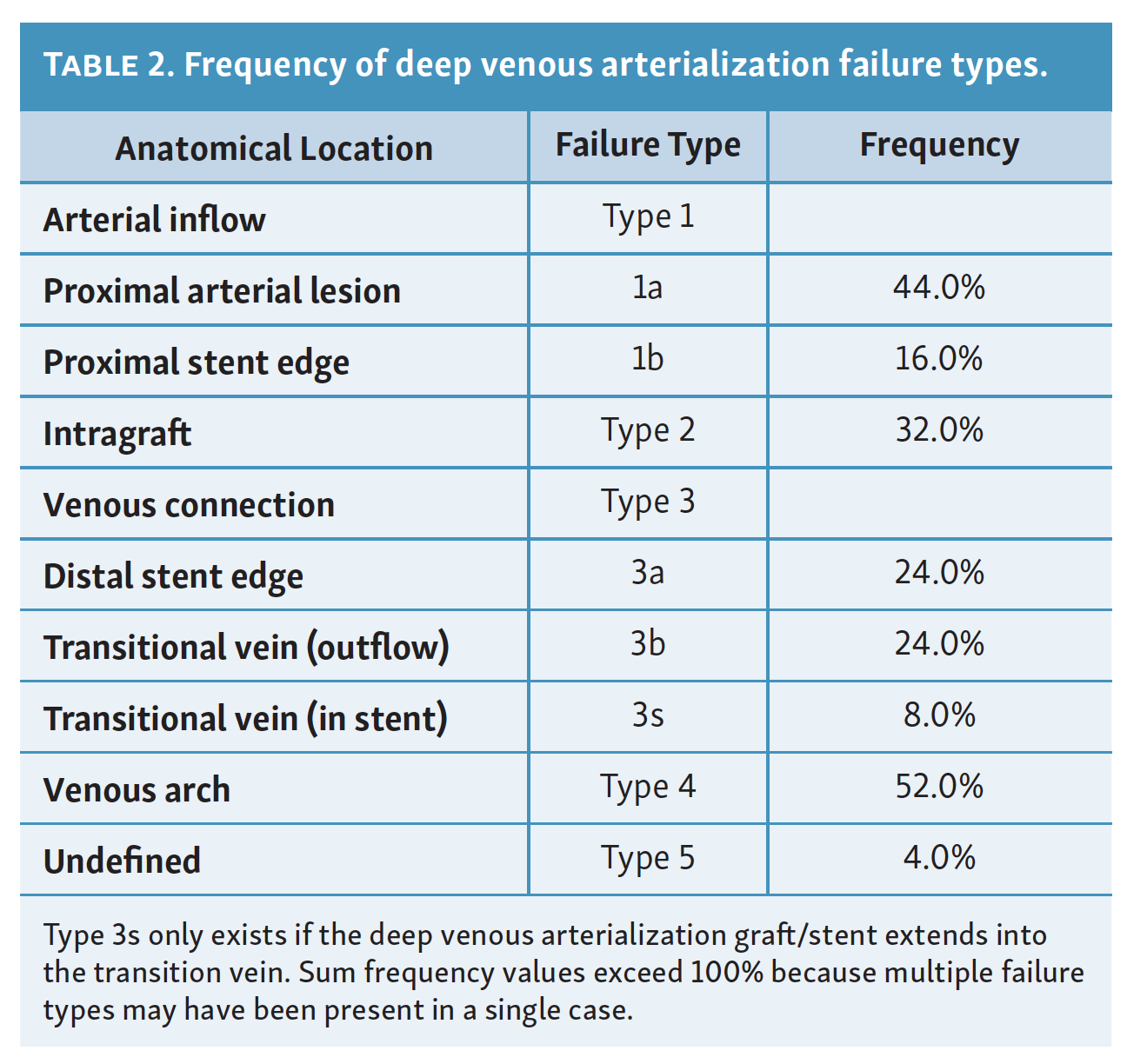
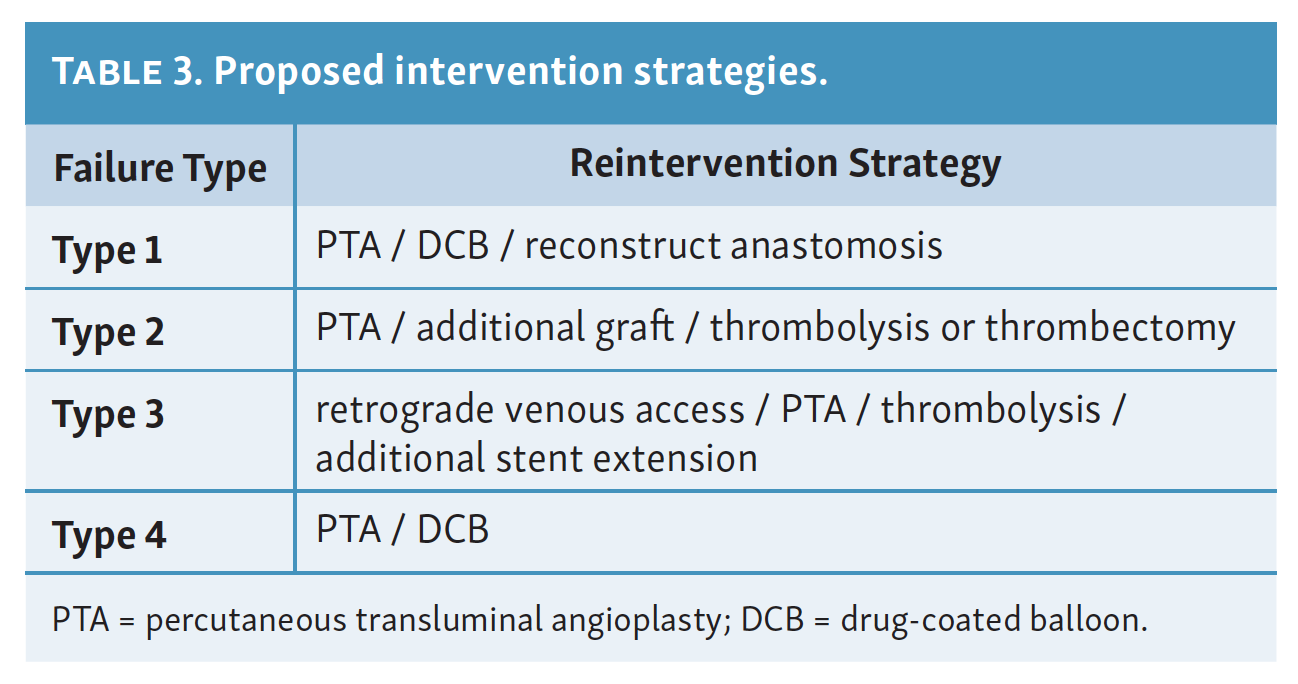
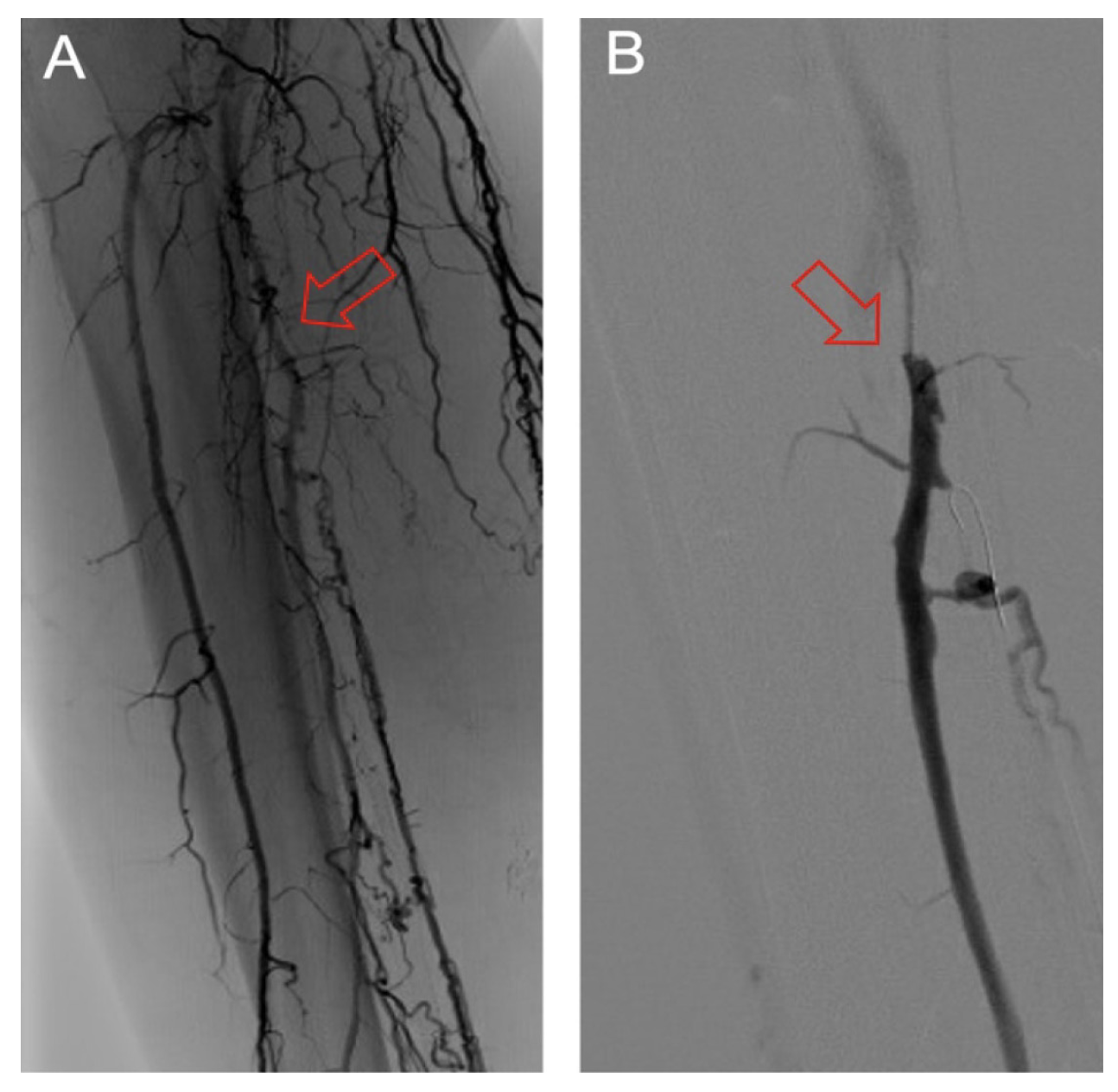
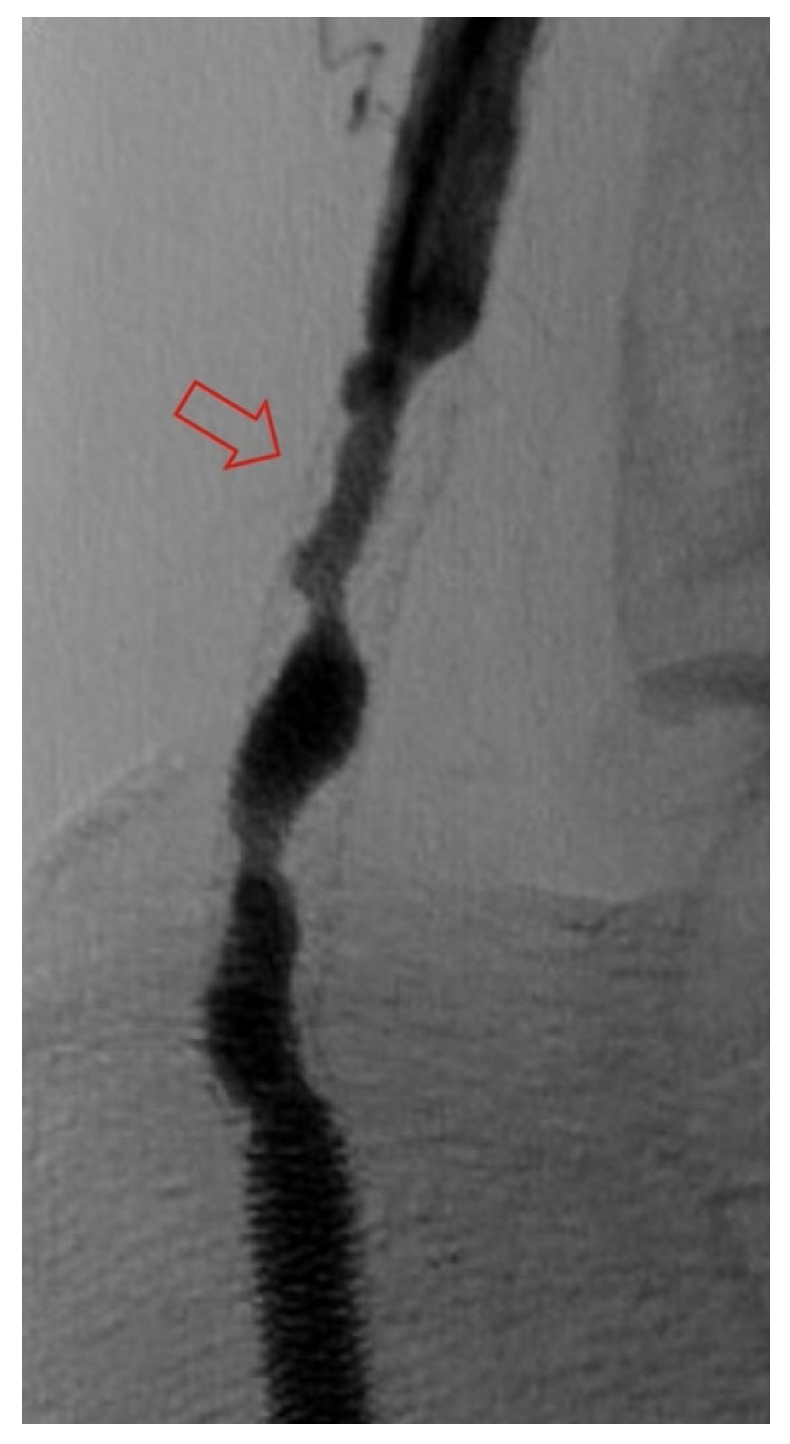
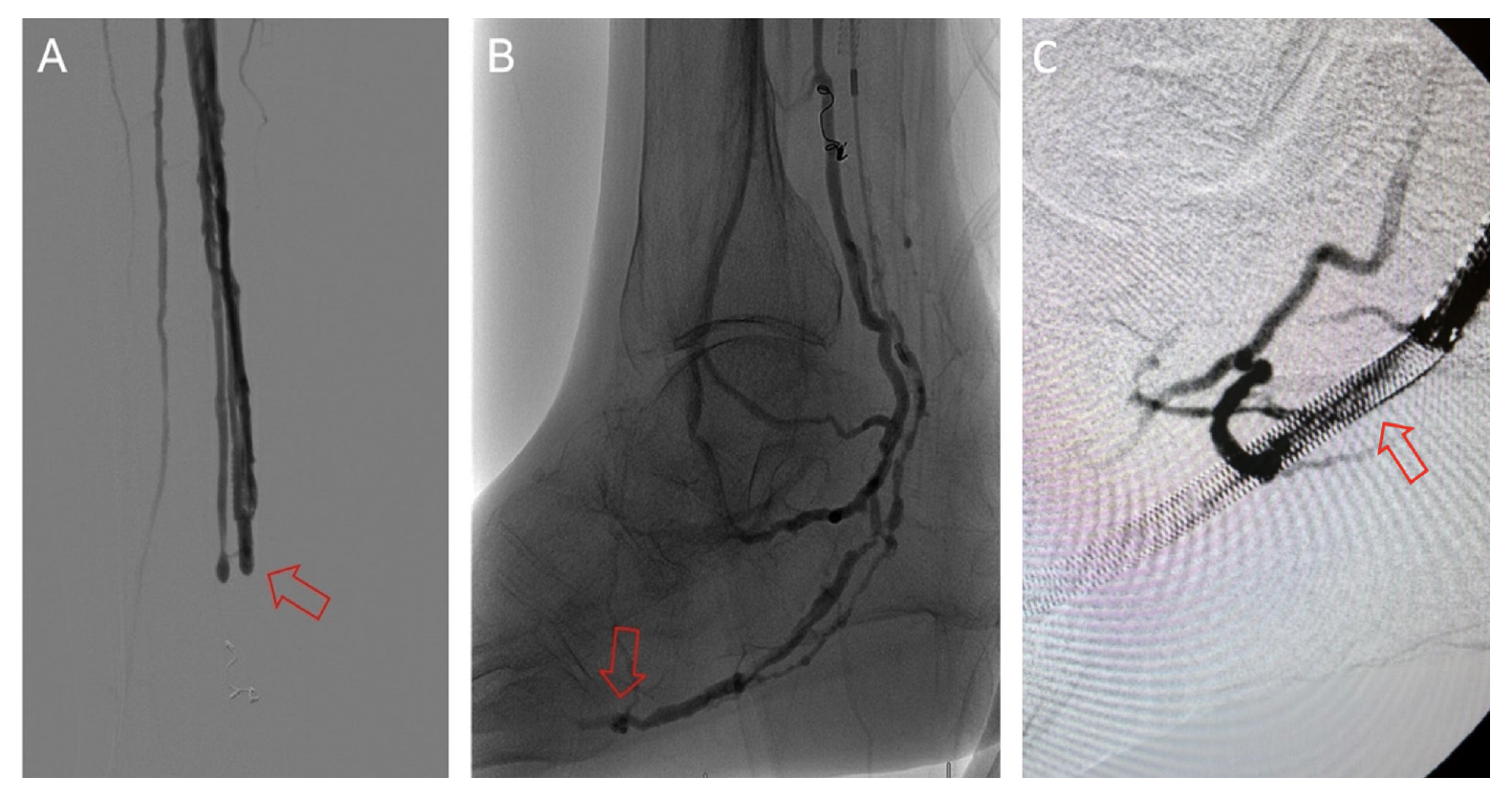
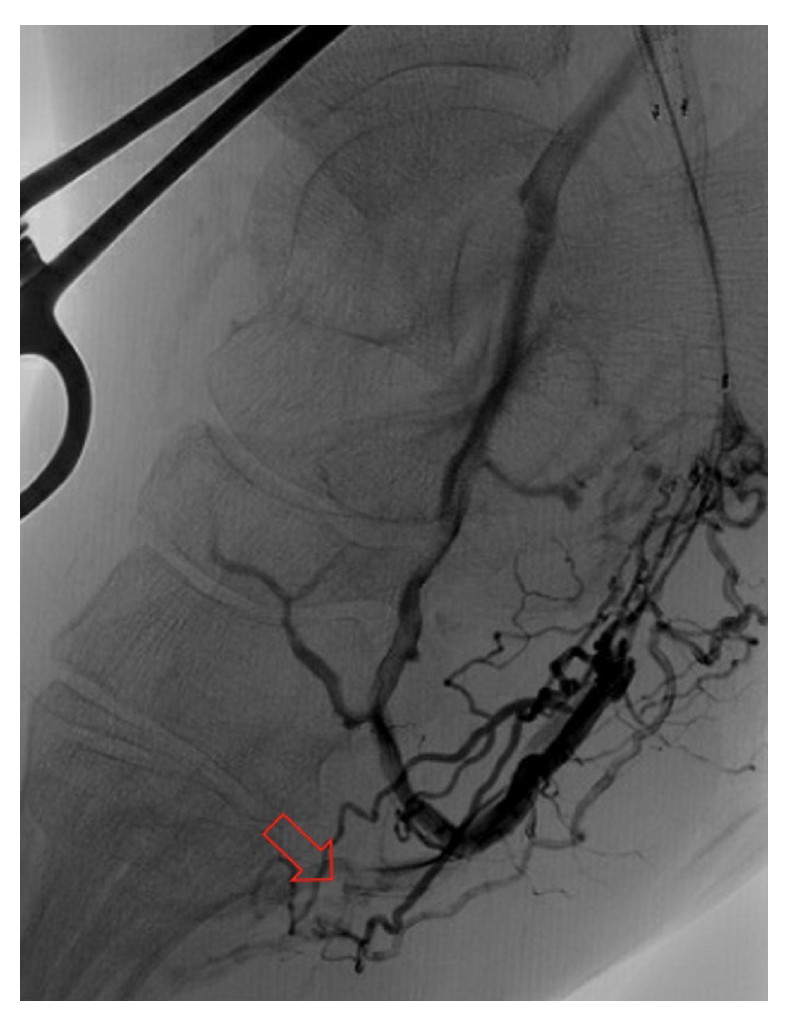
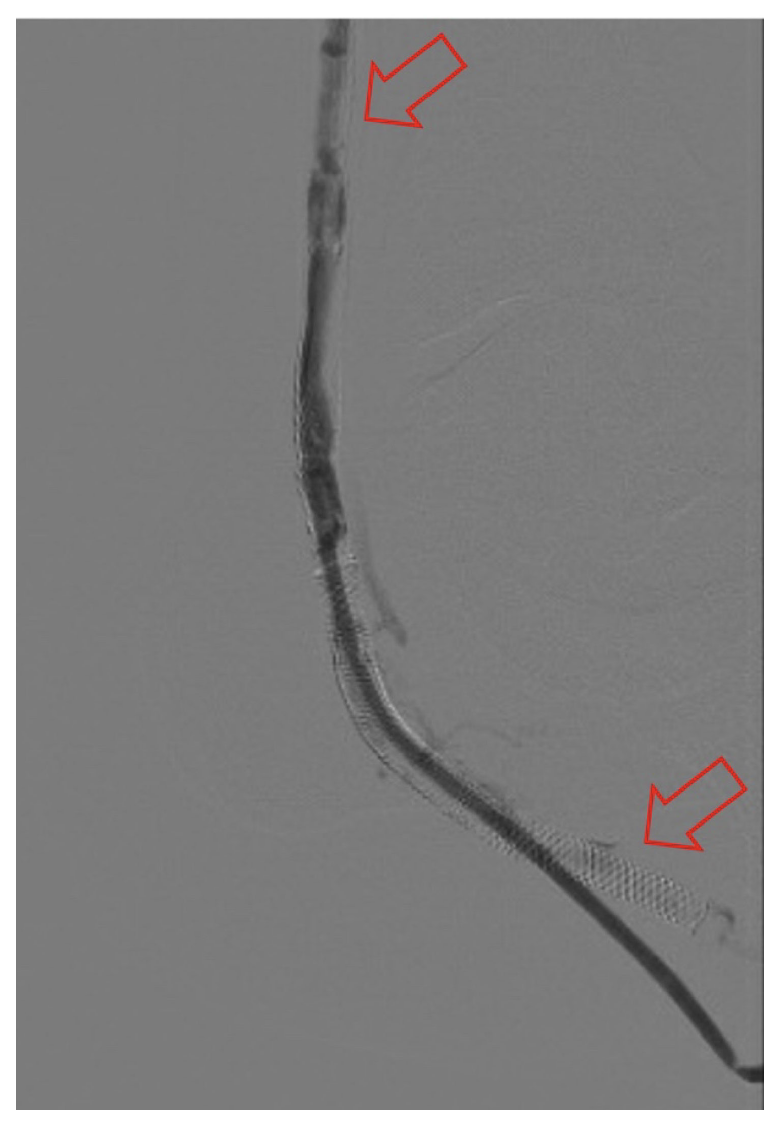
Discussion
Clinically driven DVA reintervention rates are common, with 37% of cases in this experience requiring a clinically driven repeat procedure. The most common DVA failure types present in this cohort were Type 1a and Type 4, which were present at a frequency of 44.0% and 52.0%, respectively. Distinct and isolated patterns were identified in only 32.0% of patients, whereas the majority of patients presented with a combination of angiographic failure patterns.
The PROMISE I trial found that 16 of 31 successfully treated patients (52%) required reintervention, with 75% of these reinterventions involving the arterial inflow tract.7 The ALPS study found that approximately 67% of patients suffered an occlusion of the DVA within a median time of 2.6 months, with 54.8% of all patients requiring some reintervention, especially concerning venous outflow.6 Within our cohort, 37% of patients underwent reintervention, which is lower than these earlier reports, although the decision making regarding clinically necessary reintervention may vary with different operators. As with both the Promise I and ALPS studies, we identified reinterventions to be predominantly due to combinations of arterial inflow and venous arch failures. While restenosis of previously treated arterial lesions is a well-described event, patency loss due to venous lesions is unique to DVAs. The etiology of these venous stenoses and occlusions is not defined. For transitional vein lesions, a potential cause is unrecognized, uncovered, resistant or recurrent venous valves. For these cases, the use of a dedicated valvulotome may confer some patency benefit. However, many transitional vein (Type 3b) failures were diffuse. This finding, along with frequent reocclusion of the revascularized pedal venous arch, suggests an aggressive process of intimal hyperplasia, possibly due to arterialization of these venous structures similar to what has been observed in hemodialysis fistulas.9 In addition, in our experience, the foot veins often demonstrated baseline obstructions prior to DVA creation, implying that longstanding nonreconstructible arterial disease may be associated with or causative of pedal venous disease as well. Some operators will coil embolize the proximal venous drainage pathways in an attempt to augment forward flow into the pedal venous arch, but it is not clear if this strategy would improve patency. Additional data will be needed to further evaluate and validate these findings.
Recognizing causes of DVA failures in our study had implications for management. Type 1 failure patterns were approached with plain old balloon or DCB angioplasty. Retrograde access into the DVA stent-graft conduit facilitated these procedures and allowed recreation of the proximal arterial-venous connection if an occlusion at this location could not be traversed with a wire. Type 2 failures (Figure 3) were treated with PTA and/or placement of an additional stent-graft to cover resistant lesions. Type 3 lesions (Figure 4) were managed with PTA, caudal extension of the original stent-graft, or use of a Supera woven nitinol bare-metal stent in the transitional vein. Notably, crossing the distal stent edge and transitional vein lesions with an antegrade approach was not possible in some cases, necessitating repeat retrograde venous pedal puncture for bidirectional crossing and wire rendezvous to complete the reintervention successfully. As a result of this experience, we routinely prep the foot for all reinterventions and quickly adopt a retrograde approach if initial wire crossing is a challenge. Type 4 (Figure 5) are addressed with PTA or DCB, although venous arch reocclusion can be difficult to traverse with catheter-wire techniques, and in 1 case resulted in a failure to restore DVA flow. For cases with combinations of failure types, multiple treatment modes may be indicated based upon each lesion type (Figure 6).
Study limitations. Limitations of our study include its retrospective nature and inability to compare outcomes with a control group. The relatively low number of reinterventions and skew of failure types prevents a validation of the best treatment approaches for each failure type. Further studies will be needed to confirm these findings.
Conclusion
DVA failures and reinterventions are common. Venous lesions are more common in DVA failure and can result in end-of-life for the DVA conduit. It is possible to define patterns of restenosis and use this to guide treatment plans, as proposed by our model. Further studies examining the application of these proposed classifications and intervention strategies in a larger population would be beneficial in confirming the validity of this model.
Disclosures
From the 1Keck School of Medicine of the University of Southern California, Los Angeles, California; 2Advanced Intervention and Vascular Services, Teaneck, New Jersey; and 3University of New England College of Osteopathic Medicine, Biddeford, Maine.
Disclosure: The authors have completed and returned the ICMJE Form for Disclosure of Potential Conflicts of Interest. The authors report no conflicts of interest regarding the content herein.
The authors report that patient consent was provided for publication of the images used herein.
Manuscript accepted July 25, 2022.
Address for correspondence: John H. Rundback, MD, Advanced Intervention and Vascular Services, Interventional Institute, Holy Name Medical Center, 718 Teaneck Rd, Teaneck, NJ 07666. Email: johnrundback@gmail.com